Our group focuses
on unveiling the many mysteries on core-collapse supernovae and gamma
ray bursts (CC-SNe & GRBs) from a theoretical point of view. CC-SNe
& GRBs are believed to be the
most powerful explosions in the universe, and yet very little about their explosion mechanisms
are known to mankind. Members of our group are fighting hard to understand
these extreme phenomena using advanced physics and numerical techniques
in our arsenal. In many cases, we seek power from the quickly
advancing
supercomputing resources in Japan to make progress in our
investigations. These 'astrophysical big bangs' (from the central
engine to their remnants) continue to fascinate us with puzzling
astronomical phenomena in every scale (e.g. gravitational waves,
r-process nucleosynthesis, particle acceleration, high-energy
neutrinos, ultra high-energy cosmic rays, gamma-ray emission, etc), which can be
understood only by a full use of advanced physics. We are
attacking all these problems simultaneously so that we will understand
the fuller picture of the most powerful explosions in the universe. |
Supernova explosions and Nucleosynthesis
I) Explosive nucleosynthesis in core collapse SNe
Click to enlarge
|
Core-collapse
supernovae (CCSNe), the death of massive stars, are one of the most
drastic
explosions in the Universe. However, the mechanism of CCSNe has not
been understood theoretically even by recent supercomputing efforts.
Multi-dimensional effects would be the keys ot understand the
mechanism. Therefore, 3-D simulations of CCSNe are highly desired
although they are typically very challenging. For example,
hydrodynamical instabilities affect not only the feasibility of the
explosion but also the mixing of the ejected matter, which is partly
composed of newly-synthesized heavy elements (e.g., radioactive56Ni and
even heavier metals) born in the explosive nucleosynthesis. Actually,
observations of SN1987A (the most frequently observed SN) imply that
some 56Ni synthesized is conveyed into outer layer of the star by the
mixing in contrast with sample spherical (i.e. 1-D) estimations.
Most of previous simulations considering the hydrodynamc mixing in
core-collapse supernova envelops failed to reproduce fast moving 56Ni
beyond 2000 km/s, as suggested by observations of SN1987A. However in
these simulations the large scale asymmetries, which are generated
naturally from hydrodynamic instabilities during the explosion phase of
core-collapse supernova, are often neglected. The explosion is usually
assumed to be spherically symmetric, and small scale perturbations are
added artificially after the explosion to break the symmetry. The
presence of the large scale asymmetries should help facilitating mixing
processes to convey 56Ni to the outer layers of the exploding star.
Thus, our group is interested in performing state-of-the-art long-time
3D core-collapse supernova simulations which follow the complete time
evolution from the onset of the explosion until late times. These
simulations are extremely computationally challenging because vast
length- and timescales must be resolved in the simulations. However,
recent development in high performance computing has made these
simulations become feasible.
CCSNe play an important role as factories of heavy elements, e.g., "Gold". If
rapid neutron capture process (r-process) occurs in CCSNe, heavy
elements including Gold could be synthesized. However, the specific
type(s) of CCSNe that can realize the r-process, or whether CCSNe are
resposible for the creation of r-process elements in the first place
are not clear. Recently, the Radioactive Isotope Beam Factory (RIBF)
facility in RIKEN are starting to unveil the important features of
unstable nuclei related to r-process. Our group tries to figure out the
mechanism of CCSNe and the origin of heavy elements using
state-of-the-art numerical simulations and the most up-to-date
experimental results obtained by RIBF.
II) Emission of Supernovae and radiative transfer
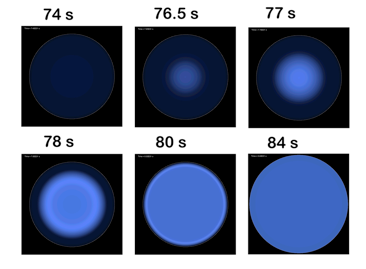
The calculated dynamics of the outburst of a star (shock breakout and supernova), as if it would be visible to an observer.
|
Phenomenon
of supernovae in most cases should start with a bright flash,
caused by a shock wave emerging from the surface of the progenitor
stars after the phase of collapse of thermonuclear explosion in their
interiors.
The detection of such outbursts associated with the supernova shock
breakout can be used to obtain information about the explosion
properties and pre-supernova parameters, which is necessary to
understand the physical processes that underlie this phenomenon.
Study of supernova shock breakouts has become particularly timely owing
to the recent detections of this phenomenon by the SWIFT and GALEX
missions. The number of future/ongoing wide and/or deep-field surveys
capable of observing shock breakouts is also increasing dramatically,
including PTF, LOSS, CRTS, KWFC, Skymapper, DES, Pan-STARS and
Subaru/HSC, LSST.
Shock propagation at the epoch of shock breakout cannot be considered
as adiabatic, which makes it difficult to construct analytical
solutions. This necessitates the usage of numerical calculations of the
process in which radiative transport plays a very important role. Our
group approaches this problem using multi-group radiation hydrodynamics
codes STELLA and RADA that allow us to calculate shock breakout for
compact pre-supernovae and take into account a number of relativistic
effects
Supernova Remnants
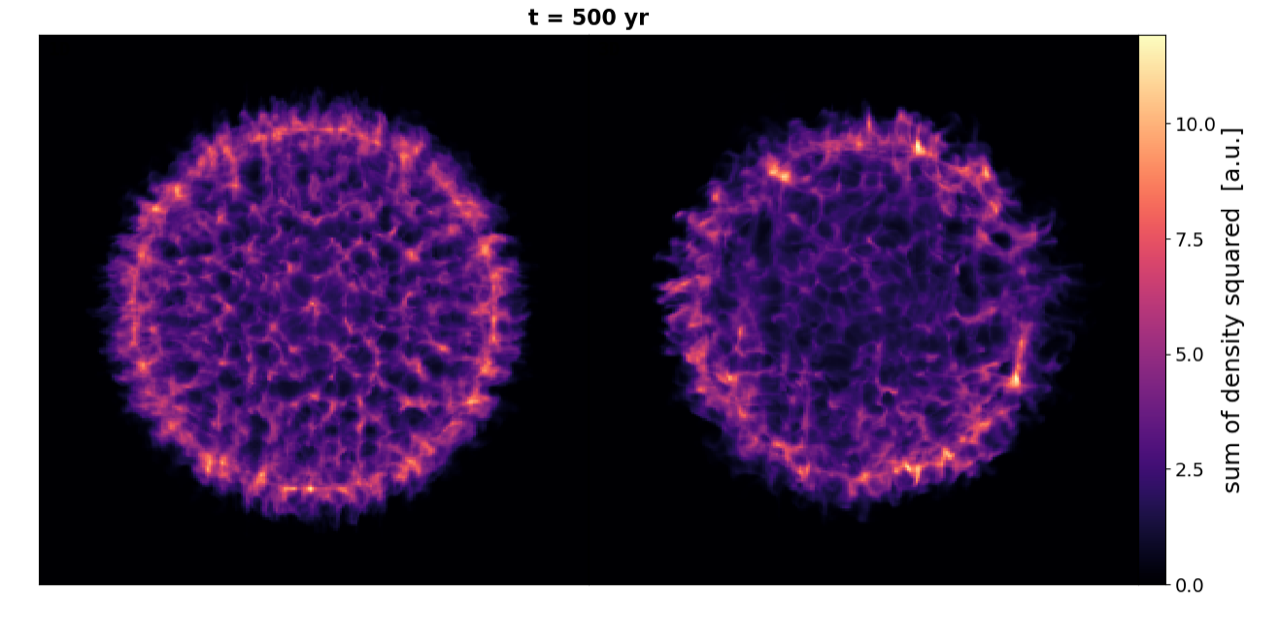
Simulated image of a SNR, as would be observed in X-rays, 500 yr after a N100 explosion. Left: starting from (effectively 1D) spherically symmetric initial conditions, right: starting from realistic (fully 3D) realistic initial conditions. The morphology of the SNR bears the imprint of the explosion.
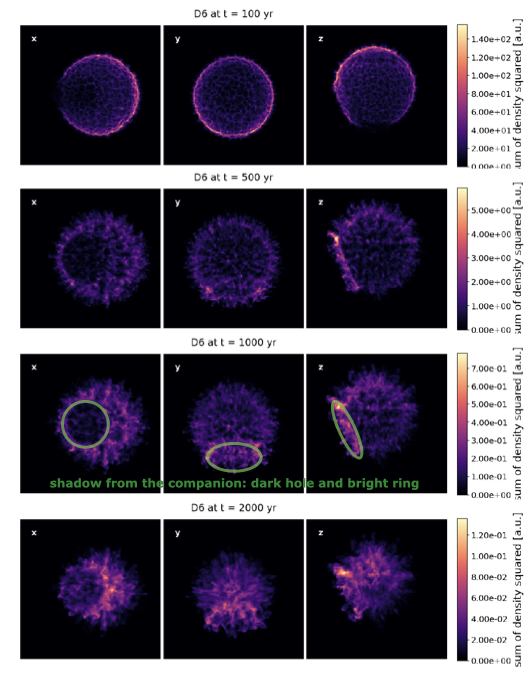
Morphology of a D6 SNR up to 2000 yr. Shown is the projection of the density squared, which is a proxy for the thermal X-ray emission in the shocked region. The presence of a surviving WD companion affects the development of the SNR up to late ages.
|
After the explosion of a star as a supernova, a supernova remnant (SNR) is formed by the interaction of stellar ejecta with the interstellar medium. Young SNRs are characterized by strong shocks that heat and ionize the gas, generate magneto-hydrodynamic turbulence, and accelerate particles to relativistic energies. They radiate at all wavelengths, especially in the X-ray domain, where spectro-imaging observations can provide a wealth of information on the shock dynamics and the explosion physics.
I) Particle acceleration and the origin of cosmic rays
Our research activities also involve the study of cosmic rays (CRs) with a
Galactic origin, especially on their very probable link with supernova
remnants (SNRs), using the latest numerical techniques and sometimes
also analysis of observation data from space telescopes. SNRs are
objects known to emit very energetic photons in X-ray and Gamma-ray,
that must be produced by local populations of high-energy particles.
Existence of strong collision-less (magnetic) shocks have been
confirmed in SNRs, which can indeed accelerate charged particle locally
to relativistic energies via the Nonlinear Diffusive Shock Acceleration
(NL-DSA) mechanism with very appreciable efficiencies. Unfortunately,
the confirmation of CR production at SNRs and their shocks
is a very nontrivial task since CRs are deflected by the magnetic field
in Milky Way and hence cannot be measured directly from their sources.
However, many SNRs can be observed
across the whole frequency spectrum, spanning from radio to TeV
gamma-ray energies. A good understanding of the multi-wavelength
emission from these cosmic accelerators is absolutely key to unravel
the long-standing puzzle of Galactic CR origin.
Because of the tight coupling between the plasma and the particles in
NL-DSA, and the wide range of space-, time- and energy-scales involved,
we mostly rely on numerical simulations, that couple hydrodynamic and
kinetic approaches. Our group performs 1-D (with micro-physics) and
global multi-D hydrodynamical simulations to study the dynamics, particle
acceleration, broadband emission and neutrino production for various
types of SNRs. Our results can be directly compared with current and
future observations from radio to TeV energies to accurately quantify
the contribution of SNRs to the production of CRs within Milky Way and
in other galaxies similar to ours. Meanwhile, we have started to tie
our code to dedicated multi-D simulations of SN explosions and
nucleosynthesis, that will provide us a consistent evolutionary picture
of the very last stages of stellar evolution and their contributions to
chemical enrichment of the interstellar space. We have been performing
simulations of the evolution and emission from young supernova remnants
in 3D, for the first time including both the development of Rayleigh-Taylor
instabilities and efficient particle acceleration. The simulations are
post-processed at a given age to compute both the thermal emission from
the shocked plasma (in the X-ray band), that is out of ionization equilibrium,
and the non-thermal emission from the accelerated particles (in the radio to
X-ray band), that depends on the fate of the magnetic field. This uniquely
enables us to produce realistic synthetic maps, as observed in projection.
This allows us to assess the impact of CRs on the morphology of the remnant.
II) From the supernova engine to the supernova remnant
Another very interesting question is to what extent and for how long the SNR bears the signature of the initial explosion. Type Ia SNe are believed to mark the thermonuclear explosion of a white dwarf (WD). Despite their importance in cosmology, their explosion mechanism is still unclear. It is unknown whether they are produced by single-degenerate progenitors, via accretion, or by double-generate progenitors, via mergers – or a mixture of both, or other mechanisms. Recent progress in the simulation of SNe has shown the importance of turbulence and asymmetries in successful explosions, which prompts us to revisit the subsequent phase, the remnant phase. Can we use the SNR morphology as a probe of the explosion mechanism? Recent work by Orlando et al has shown the interest of this approach for a core-collapse SNR like Cas A. We have been arguing for the case of a Type Ia SNR like Tycho.
First, we have run 3D simulations of a SNR starting from the output of a 3D simulation of a canonical SN model, the thermonuclear explosion of a Chandrasekhar-mass carbon-oxygen WD (model N100). By analyzing the wavefronts we were able to quantify the imprint of the explosion on the remnant over time. Assuming a uniform ambient medium, we found that the impact of the SN on the SNR may still be visible after hundreds of years. The newly simulated maps look more realistic than in previous works based on spherically symmetric ejecta profiles.
Second, we have made a comparative study of four different explosion models, still for a single WD of Chandrasekhar-mass, but with different levels of asymmetry. These were obtained by varying the number of ignition points (N100 vs N5) and the propagation of the flame (ddt = deflagration to detonation transition, def = pure deflagration). We observed that N100 models produce different remnants than N5 models: the latter produce asymmetric remnants, an asymmetric shell for N5ddt, a regular but off-set shell for N5def; and that ddt models produce different remnants than def models: the latter show a peaked remnant at the centre and large-scale plumes at the edge.
Third, we considered a different kind of model, where the primary WD explodes via a double detonation, despite being of sub-Chandrasekhar mass, while the secondary WD survives and is ejected away. This has been called a dynamically-driven double-degenerate double-detonation or D6. We simulated in 3D for the first time the evolution of a D6 SNR for thousands of years after the explosion. Assuming a uniform ambient medium, we revealed specific signatures of the progenitor system and explosion mechanism. In particular the companion WD produces a large and lasting conical shadow in the ejecta.
Our work shows the intrinsic diversity of thermonuclear SNe and their remnants, and offers new perspectives for the interpretation of observations of young nearby SNRs. The combination of 3D simulations and spatially resolved spectroscopic observations of SNRs will enable us to better constrain explosion mechanism(s).
Recent publications
- Ferrand, Warren, Ono, Nagataki, Röpke, Seitenzahl, “From supernova to supernova remnant: the three-dimensional imprint of a thermonuclear explosion”, ApJ, 877, 136, 2019
- Ferrand, Warren, Ono, Nagataki, Röpke, Seitenzahl, Lach, Iwasaki, Sato, “From Supernova to Supernova Remnant: comparison of thermonuclear explosion models”, ApJ, 906, id. 93, 2021
- Ferrand, Tanikawa, Warren, Nagataki, Safi-Harb, Decourchelle, “The double detonation of a double degenerate system, from Type Ia supernova explosion to its supernova remnant”, ApJ, 930, id. 92, 2022
- Online gallery of interactive 3D models (link to Ferrand's personal page)
Neutron Star Mergers and r-process Nucleosynthesis
Combined abundance pattern (mass fraction as function of mass number) of elements synthesized in
outflows of binary neutron-star and neutron-star black-hole mergers and their remnants as resulting
in some of our simulations (colored symbols). The remarkable similarity to the r-process pattern
observed in our Sun (black circles) suggests neutron-star mergers as a possible source of these
elements.
|
The mergers of two neutron stars or of a neutron star with a black hole are spectacular and very energetic events that have been intensively studied already for several decades, even despite the lack of a single direct observation up to the present day. However, after the recent discovery of binary black-hole mergers by the gravitational-wave detector LIGO, and therefore the first direct proof of the existence of gravitational waves, there is ample reason to expect also the first observations of neutron-star mergers very soon. One of the aspects that makes these events so fascinating and that is studied in our group is that during and after a neutron-star merger a substantial amount of matter from the newly disrupted neutron star(s) can be expelled into the interstellar medium. If this outflow material is massive enough and has a sufficiently high neutron density, the rapid-neutron-capture (r-) process could be activated. This, in turn, could mean that not (only) core-collapse supernovae but (also) neutron-star mergers are siginificant sources of the heaviest elements in our Universe. Another long-standing puzzle connected with neutron-star mergers is represented by the question if these events are viable central engines of short gamma-ray burst (sGRB), which are regularly observed by telescopes but whose origin is still unidentified. We study these questions by means of neutrino-hydrodynamics simulations, i.e. by solving numerically the time-dependent equations describing the motion of the neutron-star fluid and the transport of neutrinos. The latter type of equations are numerically cumbersome but are of major importance to properly follow the composition and energetic properties of the remnant of the neutron-star merger.
Explosion Mechanism of Gamma-ray Bursts
I) 3-D general-relativistic magnetohydrodynamics
An
example of 3-D GRMHD simulation on formation of a relativistic jet
using YAMATO code The rapidly rotating black hoke is driving a
relativistic jet with a help of magnetic fields (white curves). Colors
represent density contours.
|
It
is an observational fact that some gamma-ray bursts (GRBs) happen
together with CC-SNe. The explosion energy of these supernovae can be
ten times larger than that of normal core-collapse supernovae.
Therefore, the central engine of GRBs should be entirely different from
normal core-collapse supernovae, although its detailed mechanism is
still poorly understood. We are challenging ourselves to understand the
explosion mechanism with help of super-computing power, the developing
the state-of-the-art General Relativisitic Magneto-Hydro Dynamic
(GRMHD) code, a.k.a. the YAMATO code
(YAMATO "大和" is the histric
name of "JAPAN").
We
are particularly interested in the mechanism of energy extraction from
a rotating black hole which is expected to form as a result of the
core-collapse of a rapidly rotating massive star. In principle,
according to general relativity, it is possible to extract rotational
energy from a rotating black hole. We are investigating whether this
effect is self-sufficient to drive the most powerful explosions in the
Universe (GRBs + CC-SNE).
Recent publications
- S. Nagataki, "Rotating BHs as Central Engine of Long GRBs: Faster is Better", PASJ 63, 1243-1249, 2011
- S.
Nagataki, "Development of General Relativistic Magnetohydrodynamic Code
and its Application to Central Engine of Long Gamma-Ray
Bursts", ApJ 704-950, 2009
II) GRB jet propagation in progenitor stars
A
grand challenge in the GRB jet is to construct a self-consistent theory
that is responsible for the generation, acceleration, and collimation
of the relativistic jet. The interaction of the relativistic jet with
the progenitor star and interstellar
medium is very important to reveal global dynamics and structure of the
GRB jet. The hydrodynamical structure of the relativistic jet is also
important in order to produce GRB emission. Our group investigates the
physics of the propagation of the relativistic jet in the progenitor
star through
3-D numerical simulations. The basic property of the propagation of the
relativistic jet through the ambient medium is useful for understanding
the jet dynamics in other systems such as AGNs and microquasars.
III) Multi-fluid simulations of relativistic outflows
It is now well recognized that magnetic fields play a very important role
in many astrophysical phenomena and in particular in those involving
relativistic outflows. The magnetic fields are likely to be involved in
launching, powering and collimation of such outflows. The dynamics of
relativistic magnetized plasma can be studied using diverse
mathematical frameworks. The most developed one so far is the single
fluid ideal relativistic Magnetohydrodynamics (RMHD). The framework of
resistive RMHD allows to incorporate this magnetic dissipation but the
inevitably phenomenological nature of its Ohm's law puts constraints on
its robustness. At the other extreme is the particle dynamics,
describling the motion of individual charges in their collective
electromagnetic field. The numerical stability considerations require
particle-in-cell (PIC) codes to resolve the scales of plasma
oscillations. The accuracy considerations can be
even
more demanding, pushing toward the particle gyration
scales.
Somewhere
in between lies the multi-fluid approximation, where plasma is modeled
as a collection of several inter-penetrating charged and neutral
fluids, coupled via macroscopic electromagnetic field and inter-fluid
friction.
Undoubtedly, this approach is not as comprehensive in capturing the
microphysics of collisionless plasma as the particle dynamics (and
kinetics) simulations. However, it does this better than the
single-fluid MHD treatment. The ability of two-fluid approach to
decribe accurately the magnetic reconnection phenomenon is probably the
most important advacate for this method. The inter-fluid friction term
is analogous to resistivity. In the absence of extact analytic
solutions of equations with non-vanishing inter-fluid friction term, we
are force to try problems for which this term is expected to be of
critical significance. This important problem merits a comprehensive
study, which we are planning to carry out in the near future.
Recent publications
- Barkov M, Komissarov S., Korolev V., Zankovich A., "A
multi-dimensional numerical shecme for two-fluid Relativistic MHD", MNRAS, 438, 704-716, 2014
Emission Mechanisms of Gamma-ray Bursts
I) Photospheric emission and Monte Carlo simulations
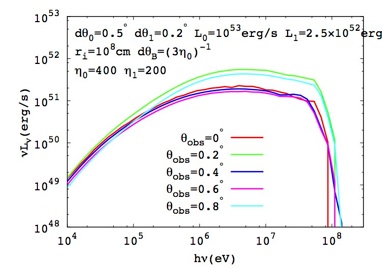
Example
of emission spectrum from a relativistic jet obtained by performing a
Monte Carlo simulation of radiative transfer. Jets having a stratified
structure can successfully reproduce the typical spectrum of GRBs.
|
Gamma-ray
burst is the most luminous phenomena in the Universe. It is believed to
be induced by an ultra-relativistic jets originating from a compact
objects. However, exactly how the gamma-rays are produced within the
jet is still under hot debates. Recently, the so-called "photospheric
emission model" is considered to be one of the most promissing
candidates for GRB emission mechanism. In this model, the gamma-rays
are emitted from the jet when it becomes transparent to radiation.
Since the interaction with the jet material plays an important role in
determining the properties of the emitted gamma-rays, detailed
radiative
transfer calculations are essential to model the GRB phorospheric
emission.
In
our group, we approach this problem by performing Monte Carlo
simulations that solve the propagation of gamma-rays within the jet. We
are particularly interested in how the hydrodynamical structure of the
GRB jet influences the properties of the resultant emission, including
its spectrum, polarization and light curves. Starting from a series of
toy models of jet structures, we are investigating the conditions udner
which the photosheric emission can successfully reproduce the observed
characteristics of GRBs.
II) Jitter radiation model
Explorations
of physical processes related to random and small-scale magnetic fields
are exciting and can be highly related
to GRB emission. Jitter radiation, which involves relativistic
electrons radiating in random and small-scale magnetic fields, can be
applied to explain high-energy emission of AGNs and GRBs. We also
explore the possibility that high polarization degree discovered in
some GRBs in high-energy can be reproduced by the jitter polarization
process. Compton scattering of jitter photons can be also applied to
explain the GeV emission of some special classes of GRBs.
III) Novel radiation mechanisms
Our
group also investigate more general radiation mechanisms than the
jitter radiation. In the emission regions of GRBs, we can expect
turbulent electromagnetic fields which are
generated by some plasma instabilities. The radiation spectra from
accelerated electrons in such turbulences have not been fully
investigated. We employ first principle numerical method to clarify
them. We have found novel emission spectrum in the intermediate regime
between synchrotron regime and jitter regime. Also, we have clarify the
general properties of radiation spectra for the Langmuir turbulences.
The radiation properties for more general turbulences are going to be
investigated.
IV) Optical observations of GRBs
Follow-up
observation of GRBs is another interesting research topic carried out
in our group. Multi-wavelength observations are more than essential to
understand the mysterious phenomenon of GRBs. We regularly perform
ground-based optical follow-up observations of GRBs detected in X-ray,
mostly by
the Swift satellite. In addition, observations are forged with
theoretical explanations for GRB host galaxies in our group to revearl
the true identify of GRB central engine.
Gamma-ray Burst as Cosmological Tool
GRBs
are the farthest and the most powerful objects ever observed in the
universe and therefore can be useful probes for testing cosmological
models. To this end, it is crucial to understand if they can be
considered as standard candles (astronomical objects whose luminosity
is known or can be derived from other distance-independent
observables). At first sight, GRBs seem to be far from standard
candles, with their energies spanning over 8 orders of magnitude.
However, discovering universal relations among the observables
properties of GRBs plays a crucial role in determining
whether they can be considered standard candles and can provide
insight intor the processes responsible for GRBs.
Within
this framework, Dainotti et al. (2008) discovered a new correlation for
long GRBs between the X-ray luminosity at the end of the plateau
phase, Lx and its duration, T*a (see
figure). This correlation has been updated (Dainotti et al. 2010)
used as possible redshift estimator (Dainotti et al. 2011a), applied as
cosmological tool (Cardone et al. 2009;2010) recently corrected for
selection effects (Dainotti et al. 2013a). Caveats on the use
correlations not corrected by selection effects has been presented
(Dainotti et al. 2013b) showing how we can commit errors on the
evaluation of the cosmological parameters.
Nowadays, the challenge is the user of the intrinsic correlation to
explain the most plausible theoretical models and to apply as a
cosmological tool.
Other topics
I) Ultra high-energy cosmic rays
We
are also interested in the origin(s) of ultra high-energy cosmic rays
(UHECR) and high-energy neutrinos, cosmic particles that are often
thought to come from extra-galactic space at extreme distances. Along
with construction of theoretical
models, we also try to find clues from data gathered by the latest
astro-particle experiments. Several individuals of our team are team
members of Telescope Array at Utah that studies very high-energy CRs hitting our
atmosphere from outer space.
The members contribute to the operation of the Telescope Array (TA) detectors. TA found evidence for a cluster of the cosmic rays with energies greater than 57 EeV. To accelerate the data collection speed for elucidating this new prospect, the TAx4 experiment was started. Recently our member contributed to the design, construction, calibration and the data acquisition of the surface detectors of the TAx4 experiment. The surface detectors of the TAx4 experiment started to collect cosmic ray events from 2019. We are analyzing the data to study the origins of the events.
UHECR nuclei are expected to interact with the background photons in intergalactic space. The photo-absorption of UHECR nuclei can be an important process to interpret the observables such as energy spectra and compositions of UHECRs. The Photo-Absorption of Nuclei and Decay Observation for Reactions in Astrophysics (PANDORA) project is a joint project among three experimental facilities with nuclear theories and astrophysical simulations. Some members are involved in this project and study the impact of the measurements and nuclear theories on the interpretation of UHECRs.
Currently,
a few of us are also involved in activities of the Cherenkov Telescope
Array (CTA), a near-future international ground-based observatory for
very high energy (VHE) gamma-rays. We are performing theoretical and
numerical calculations to help the team make plans for future
observations of various high-energy astrophysical objects using CTA.
II) Pulsar
Pulsar
is a rapidly rotating and strongly magnetized neutron star which emits
radiation beam in a rotation period. The word "pulsar" is a contraction
of "pulsating star". Today, more than 2000 pulsars are detected,
some of them are one of the brightest source in our galaxy. In
addition, young pulsar is usually surrounded by luminous
synchrotron nebula e.g. Crab nebula.
For most pulsars, the activity is drived by rotationing neutron
star (Rotation powered pulsar). For simlicity, it is just a rotating
magnet, which is known as a high-power unipolar dynamo. And
therefore, the plasma in magnetosphere is highly accelerated. The
rotation energy of pulsar is released by electro-magnetic wave
(Beam) and outflow of high energy plasma (Pulsar wind). But detailed
radiation mechanism or structure of magnetosphere have been still
controversial. To solve this problem, we have been tried
global simulation for global pulsar magnetosphere.
The simulation includes inertia of accelerated plasma and pair creation
process (photon collision and magnetic pair creation).
III) Interaction of the Stellar Wind with the Pulsar
The
structure formed by the shocked winds of a massive star and a
non-accreting pulsar in a binary system suffers periodic and random
variations of orbital and non-linear dynamical origins. The
characterization of the evolution of the wind interaction region is
necessary for understanding the rich phenomenology of Galactic
Gamma-ray binaries. For the first time, we simulate in 3 dimensions the
interaction of isotropic stellar and relativistic pulsar winds along
one full orbit, on scales well beyond the binary size. Previously only
the Kelvin-Helmholtz instability, discussed in the past, we find that
the Richtmyer-Meshkov and the Rayleigh-Taylor instabilities are very
likely acting together in the shocked flow evolution. Simulations in 3
dimensions confirm that the interaction of stellar and pulsar winds
yields structures that evolve non-linearly and become strongly
entangled. The evolution is accompanied by strong kinetic energy
dissipation, rapid changes in flow orientation and speed, and turbulent
motion. The results of this work confirm our prediction for the loss of
the coherence of the whole shocked structure on large scales.
animation of the simulation
https://youtu.be/4Xu1ZHl0nkk
https://youtu.be/adtMPuERsoc
https://youtu.be/U1WOyUaPk98
A
mysterious X-ray-emitting object has been detected moving away from the
high-mass gamma-ray binary PSR B1259-63, which contains a
non-accreting pulsar and a Be star whose winds collide forming a
complex interaction structure. Given the strong eccentricity of this
binary, the interaction structure should be strongly anisotropic, which
together with the complex evolution of the shocked winds, could explain
the origin of the observed moving X-ray feature. We proposed that a
fast outflow made of a pulsar-stellar wind mixture is always present
moving away from the binary in the apastron direction, with the
injection of stellar wind occurring at orbital phases close to
periastron passage. This outflow periodically loaded with stellar wind
would move with a high speed, and likely host non-thermal activity due
to shocks, on scales similar to those of the observed moving X-ray
object. Such an outflow is thus a very good candidate to explain this
X-ray feature. This, if confirmed, would imply pulsar-to-stellar wind
thrust ratios of 0.1, and the presence of a jet-like structure on the
larger scales, up to its termination in the interstellar medium.
Recent publications
- Bosch-Ramon, Barkov, & Perucho, "Orbital evolution of colliding star and pulsar winds in 2D and 3D effects of dimensionality,
EoS, resolution, and grid size", 2015, A&A, 577, A89
- Barkov & Bosch-Ramon, "The origin of the X-ray-emitting object moving away from PSR B1259-63", 2016, MNRAS, 456, L64
IV) Type-I X-ray Burst
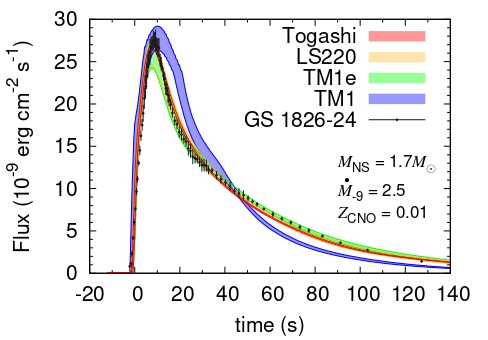
Several X-ray burst light curves with different equation of states and their comparison with the observed light curves of regular burster GS 1826-24.
|
Type-I X-ray Burst is an explosive phenomenon triggered by unstable nuclear burning in accreting neutron stars. The observed light curves involve the physics of neutron stars, their companions, and nucleosynthesis, particularly for proton-rich nuclei, and therefore we could explore many aspects of nuclear-astro physics through comparison of numerical X-ray burst models with observations. For example, nuclear equation of states in the neutron-star core, which have been still uncertain in spite of recent experimental and observational progress, are important for X-ray burst light curves, as shown in the figure. By comparing with the shape of light curves in regular X-ray bursters such as GS 1826-24, we can constrain the equation of states of neutron star states as shown in the attached figure. Thus, we are tackling X-ray burst modelings (not limited to regular bursters) and applying them to extract various uncertain information on nuclear-astro physics.
Recent publications
- Akira Dohi, Nobuya Nishimura, Masa-aki Hashimoto, Yasuhide Matsuo, Tsuneo Noda, and Shigehiro Nagataki, "Effects of Nuclear Equation of State on Type-I X-ray Bursts: Interpretation of the X-ray Bursts from GS 1826-24", ApJ 923, 64, 2021
- Akira Dohi, Nobuya Nishimura, Hajime Sotani, Tsuneo Noda, Helei Liu, Shigehiro Nagataki, and Masa-aki Hashimoto, "Impacts of the direct URCA and Superfluidity inside a Neutron Star on Type-I X-Ray Bursts and X-Ray Superbursts", ApJ 937, 124, 2022
|